Crystal Chemistry
THE NATURE OF CHEMICAL ELEMENTS
Each Mineral composed of element in various combination
elements composed fundamental building block of matter: Proton, Neutron, & Electron
Mass of p⁺ = Mass of neutron > Mass of e-
P⁺ & n held together in nucleus by attractive force
Atomic number (Z): Each element has specific number of p⁺ in nucleus & Z is number of Proton
Number of n = Number of p⁺ for elements with small Atomic number, & Number of n > Number of p⁺ for elements with higher Atomic number
تتساوى اعداد النيوترونات مع البروتونات بالعناصر الخفيفة وتزداد بالعناصر الثقيلة: لان كلما زاد عدد البروتونات تزداد قوى التنافر بينها (عدم استقرار النواة) فيزداد عدد النيوترونات لتصل الذرة للاستقرار وكلما زاد عدد النيوترونات يزداد العدد الكتلي وتنتج العناصر الثقيلة “قابلة للانشطار”
Isotope: element has different number of n
mass number : sum of number of n & p⁺
atomic mass : mass of atom divided by one-twelfth mass of ¹²C atom
Except for ¹²C atomic mass is just trifle different from mass number, Because each isotope has different number of n so each isotope must have different atomic mass
atomic weight: average weight of atomic masses of isotopes, depends on isotopic composition
Only 83 elements are available to make minerals: from 112 element in periodic table only 94 occur naturally, 11 of 94 are geologically ephemeral (occur in small amounts as short-lived radioactive isotopes produced by n-capture or radioactive decay & so it decay rapidly)
عناصر لا تدخل بتركيب المعادن (الغازات النبيلة العناصر النشطة والمصنعة بالمختبرات) والعناصر النشطة لا تدخل لان فترة وجودها صغيرة فهي تتحول لعناصر اخرى ضمن سلسلة من التفاعلات
ELECTRONS
In uncharged atom: number of e- = number of p⁺
Electron don’t orbit randomly around nucleus, but systematically organized into energy level with 4 quantum number
Pauli Exclusion Principle: no 2e- can have same of 4 quantum number
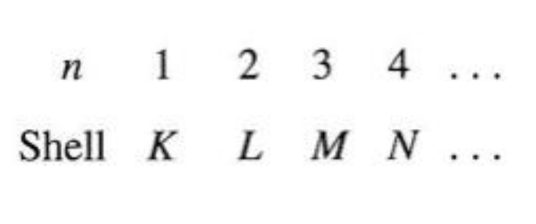
يصف مستويات الطاقة والبعد عن النواة
have any +ve integer value
energy of e- depend on η
higher η → higher energy Because higher energy associated with greater distance from nucleus
η are correlated with shells that traditionally are identified with letters (K, L, M, N…etc)
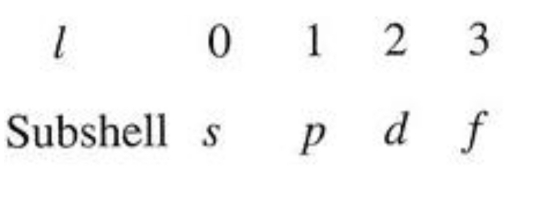
Depend on value of η : L = [0, (n – 1)]
distinguishes subshells with different shapes يصف شكل المدار
Calculation
maximum subshell number within shell is limited to n – 1
For K shell: l = η – 1 = 0 →(l=0) has 1s
For L shell: l = 2 – 1 = 1 → (l=1) 2s & 2p
subshell identified with η of shell & letter of subshell
Ex. p subshell in (n=2) is 2p

magnetic quantum number (Mι)
Depend on value of l : Mι = [-l,+l]
number of orbitals within subshell = 2l + l
It distinguishes among different orbitals with different orientations within subshell
يصف اتجاه المدارات
Spin quantum number (Ms)
Each orbital contain 2e- distinguished by Ms, & the Values of Ms = +½ or ½
electron behave spinning (magnet) on axis, so Ms taken to indicate spin to right & left
each spinning charged e- behave like simple magnet with north & south pole Because magnetic field generated by movement of e-
If electron spins are balanced, so number of right & left spins is same & no net magnetic moment formed
if e- spin aren’t balanced, net magnetic moment result
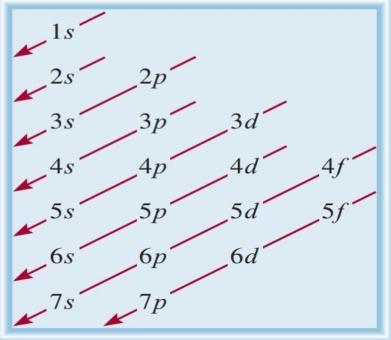
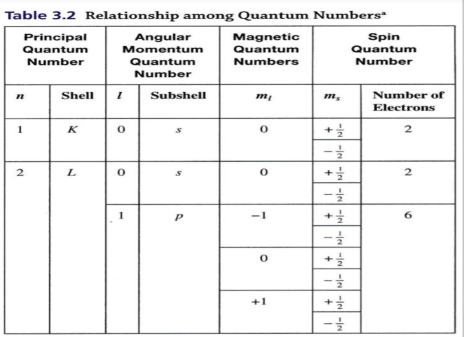
valence electrons
elements at right side of table (noble gasses) have all of their subshells filled
Core: inner shell configuration, within shells are entirely filled
Valence electrons: outer shell configuration within shells that are not entirely filled
If core is identical to electron configuration of noble gas It’s called noble-gas core
Ex, Na has Ne noble-gas core + single 3s valence electron
A pseudonoble-gas core consists of noble gas core + entirely filled d & f subshell
Ex. Arsenic (As) has pseudonoble gas core 1s, 2s,2p,3s,3p,4s, & 3d subshells + three 4p valence electrons
Formation Of Ions
Ions: atoms with excess or deficiency of e- compared to number of p⁺ in nucleus
Anions: Ion with net -ve charge because have more e- than p⁺
Cations: Ion with net +Ve charge because have fewer e- than p⁺
valence or oxidation state: charge of ion
Whether element will form anion or cation can be inferred from configuration of valence electrons
Cation Vs. Anion
Metals that have noble gas or pseudonoble gas cores, have Little energy to lose valence e- to form electron configuration of noble gas, so they form cation
Nonmetals, have valence subshells that need few e- to be filled, & nonmetals have strong affinity for e- to fill outer subshell to form anion
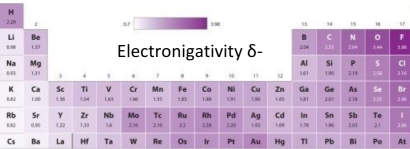
measure propensity of element to gain or lose electrons
Elements with low δ- lose outer valence e- readily to form cations
Elements with high δ- have strong affinity for extra e- & tend to form anion
δ- values are used to estimate nature of chemical bonds

ABUNDANCE OF THE ELEMENTS
8 element are present in substantial amounts (O, Si, Al, Fe, Ca, Na, K, & Mg) comprise large majority of crust, & are elements from which most common minerals are composed (all are cation except O)
The oceanic crust is richer in Fe & Mg (Basalt) than average crust
Determining composition of entire Earth is even more difficult because mantle & core cannot be sampled directly, & Estimates are obtained by:
1. Earth’s mass & density distribution as determined by geophysics
2. Composition of basalt derived from mantle & samples of mantle that arrive with magma
3. Composition of meteorites, represent material from which Earth accreted
4. Applying appropriate cosmological, geochemical, & petrophysical models to evaluate these data
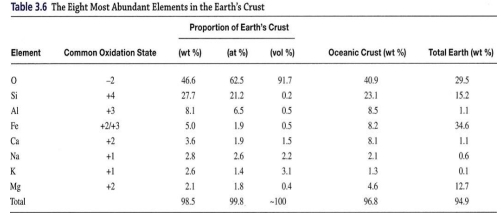
Chemical Bonds
Chemical Bonding
Is the force that hold atoms together
grouped into 2 categories:
1. bonds involve valence electrons
2. bonds don’t involve valence electrons
Some mineral have different bond types, such as Graphite:
1. Covalent σ & π bonds
2. Intermediate covalent/metallic character
3. Van Der Waals bonds
bonds involve valence electrons (Valence Related Bonding)
involve mechanisms allow elements to acquire of noble gas or pseudo-noble- gas configuration by gain, loss, or sharing valence electron, would be in lower-energy, & form stable configuration
Types of Valence Related Bonding
1. ionic bond
2. covalent bond (sigma σ or Pi π)
3. metallic bond (conduction, valence)band
Bond Not Involving Valence Electron
depend on relatively weak electrostatic forces that can develop because of asymmetric charge distribution
These bonds are sometimes referred to as molecular or intermolecular bonds
2 mechanisms by which asymmetric charge distribution is developed are illustrated by hydrogen & Van Der Waals bonding
Types of bonds don’t involve valence e-
1. van der Waals
2. hydrogen bonding
Ionic Bond
formed by electrostatic attraction between +ve & – ve ions (Anion + Cation)
Ex. halite (NaCI) make ionic bound because:
1. Exchange of electron between Na (loss) & Cl (gains) produce stable electron configuration for both
2. Na (+ve) & Cl (-ve) have opposite charges
Nature of attractive force between oppositely charged given by Coulomb law
F α (q₁* q₂) / d²
Equilibrium distance between Na & Cl is given where F = 0 (At greater distances attractive force & smaller distances repulsive force is larger) F=0 → Fattractive = Frepulsive
Charges must balance Ion bonds together if net +ve charge = net -ve charge
Ionic bond fairly strong because:
1. Ion act like charged sphere & pack together in systematic & symmetrical manner
2. +ve & -ve charges alternate to form electrical neutral crystalline solid, This represent low-energy configuration
Ionic-bonded crystals tend to be brittle
Ionic crystals have strong resistance to sliding different parts past each other Because like charges repel each other
Forcing issue result in rupture rather than ductile deformation Because structures quite orderly
Failure commonly occur along cleavage plane
Covalent Bond
formed by sharing of electrons, & occurs when orbitals of 2 atoms overlap
Provided overlapping orbitals have no more than 2 electrons combined
electrons in orbital begin move about both atom because attracted to nuclei of both atom, bond established
Strength of covalent bonds is function of degree to which orbitals of adjacent atoms overlap, & more overlap yields stronger bonds (such as in diamond)
Ionic bond not possible in diamond because:
1. all C have identical electron configuration & affinity for valence electron
2. One C cannot steal electron from another to form +ve & – ve ions
Types of covalent bond:
1. sigma (σ): has high degree of symmetry about axis parallel to length of orbitals that overlap end to end, like bond in diamond
2. pi bonds (π): orbitals overlap side to side, so e- shared laterally, found in graphite
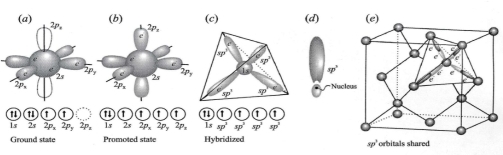
Diamond composed of (C) & noble-gas configuration obtained by gaining or losing 4e-, & To produce covalent bond it must be share 4e-, but in ground-state configuration of C only three 2p orbitals are available, 2 have e- & third has none, & 2s orbitals cannot share nay e- because contains 2e- & To create 4 unpaired orbitals, hybrid orbitals formed:
1. One of e- in 2s promoted to vacant 2p orbital; this provides 4 unpaired orbitals
2. Because all 4 bond to adjacent C must be identical, orbital in 2s & 2p hybridized into 4 identical 3sp³ orbital
3. Each of orbitals consists large lobe pointing in one direction & small in opposite, positioned around nucleus so that large lobes define corners on tetrahedron
4. Each of 3sp shared with identical hybrid orbital to form continuous crystal structure of diamond, Each are sigma σ covalent bonds
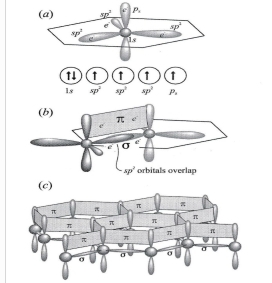
π & σ bonding within sheets of C is very strong, stronger than bonds in diamond, That attested by observation that C-C bonds in graphite are shorter than in diamond
low hardness of graphite is consequence of van der Waals bonds that hold sheets together
Metallic Bonds
considered to type of covalent bond in which valence electrons delocalized & free to move throughout crystal structure
Formation of metallic bonds depends on:
1. Valence electrons held weakly: like Metals that have low electronigativity
2. Number of electron shared must be large
3. Availability of vacant energy levels into which valence electrons can readily move: This is controlled by spacing between atoms
If spacing between atoms = infinite, so all atoms have same energy level & orbitals
Width of energy band produced by subshell increases as a proximity of atoms increases
e- become free to migrate throughout structure if:
1. energy level of unfilled band overlaps that of filled band
2. outer valence subshell is only partially filled
Mg illustrates overlap of unfilled band with filled band, When Mg atoms placed in close proximity, width of energy band increase so 3s & 3p band overlap
Abundance of available energy levels allows electrons to migrate easily via structure
conduction band band has more energy level than their electrons & can conduct electrons via crystal if voltage is applied, such ad 3p band in Mg
valence band provide electrons move into conduction band, such as 3s band in Mg
inner subshells don’t contribute to electrical conduction because energy gap, known as band gap, is present between bands
Na have conduction band + valence band
Only half of energy levels in 3s band can be occupied at any one time so remaining energy levels are available for conduction
Transition metal utilize both mechanisms to produce metallic bond, conduction occur in d band (contain unfilled orbitals) & energy level of d band overlap higher s & p bands
Metalic bond no directional because metalic bond don’t involve matching specific orbital on adjacent atoms,
Metals atoms back together in highly symmetrical manner & held together by weak bonds provided by valence e- migrating throughout structure in conductiin band
Because metalic bonds are weak metals tend to be relatively soft & malleable
Because metal atoms have their valence requirements satisfied by sea of valence e-, it very easy for one metal atom to substitute for another in crystal structure
Width of energy bands increases as atoms are brought closer together
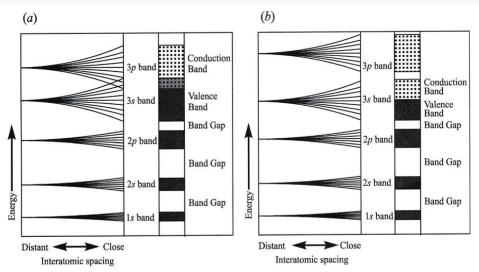
Hydrogen Bonding
Ice is most familiar mineral have H-bond
Ice composed of H₂O, Bonding occurs because H₂O polar molecule, 2H covalently bond to O by sharing electrons
Polar: Because O has high electronigativity than H, it places greater claim on shared electrons than do H & This produces electrical polarity to water molecule

Van Der Waals Bonds
Depend on asymmetrical charge distribution, but asymmetry is produced in different way
Van der Waals bonds are quite weak, & mineral have them (graphite & talc) are soft & greasy
soft & greasy properties used to advantage:
1. graphite soft & black, used to make pencil, with which sheets slide past each other
2. Talc used as body powder because it is soft & helps prevent chafing of tender skin
3. Weakness found in clay minerals also leads to major engineering problem, known as swelling soil
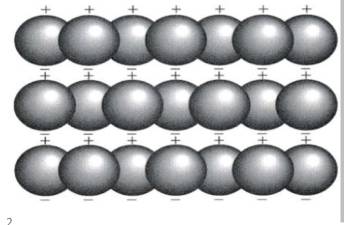
more -ve charged electron on one side than other producing polarization, & polarization on one sheet are similar in its neighborse, & Van Der Waals pond produced by weak electrostatic atraction between opposite charge of poth sheets
Relation among Valence-Dependent Bond
values of electronegativity can be used to estimate the nature of chemical bond
1. Ionic bonding should be expected if δ- is quite different
2. Covalent & Metallic involve sharing of e- among atoms with similar affinity for e- & small or zero difference in δ-
Crystals with ionic & covalent bond have low electrical conductivity because:
1. Valenc e- held tightly in specific orbital
2. Electrical conductivity increases if crystals heated because thermal energy increases
Crystals with metallic bonds have high electrical conductivity because:
1. Electron are free to migrate throughout structure in conduction bands
2. Electrical conductivity of metallic bonds decreases as temperature increases because greater thermal vibrational energy of lattice tends to impede migration of electron
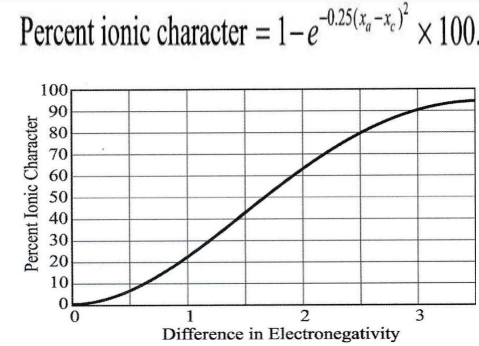
Figure show: Empirical relationship between difference in δ- & degree of ionic character produced
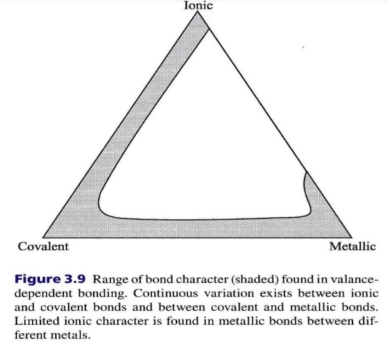
Sulfate → covalent + metallic
Silicate → covalent + ionic
Cation Vs Anion
About atoms!
Volume occupied by proton, neutron, & electron that comprise isolated atom or ion defined by probability of finding electrons at specific locations around nucleus
Probability aren’t spherical, except electron in s shells, & don’t have defined boundaries
Some probability, always exists of finding electron at great distance from nucleus
Chemical bond involve some degree of covalent bonding in which orbital from atoms or ions are inferred to overlap, to allow sharing electrons
Effective radius
Atom behave as little sphere, It convenient to define sizes of atoms in term of effective radius based on distance between centers of atoms
Effective radius of atom or ion: is size it would have if it behaved like small hard sphere
Atomic radius: effective radius of uncharged atom
Ionic radius: effective radius of anion or cation
In covalent or metallic bond bond length (L) is equal to 2 effective radius 2R
L = 2R
– Techniques of X-ray diffraction used to measure bond length & sum of effective radius of atoms
– There are 2 sets of data depending on nature of chemical bonds involved:
1. For metals & semimetals, radii based on metallic bonding in structures in which atoms closely packed so that each atom is in contact with 12 other atoms
2. For non-metals, radii based on single covalent bonds
In ionic-bond, length between anion & cation = to sum of effective ionic radii of cation Rc & anion Ra
L = Rc + Ra
Techniques of X-ray measurement length between anions & cations but cannot tell us how much of bond length to allot to cation & how much to anion
to estimate of effective ionic radii of anions & cations:
1. Comparing different structure
2. Evaluate cation-anion & anion-anion distance
principal variables influence effective ionic radius:
1. Oxidation state (valence) of ion
2. Coordination Number (CN): Number of anions in contact with cation
3. spin state: factor in transition metals with d orbitals

Oxidation State & Coordination Number
Cations are smaller than uncharged atom Increase +ve charge produce smaller ion (size decreases) because electron held more tightly& closely to nucleus
Anions are larger than uncharged atom because nucleus is less able to hold additional electrons tightly
Interatomic distances, & effective ionic radii, influenced by number of anions contact with cation (CN)
Anions larger than cations, so structure considered to consist framework of packed anions with smaller cation tucked into interstice, or hole between anions
size of interstice determine by coordination number (CN)
large hole require more anion to define boundaries
coordination number of 4 -for example- involves 4 anion arranged at corners of tetrahedron with small space in middle in which small cation may reside
as coordination number increases, effective ionic radius of cations occupying hole defined by anions
cations expand or shrink to fill space available between anions
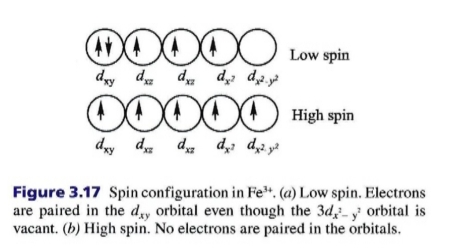
high spin state is preferred because having unpaired electrons represents lower energy configuration than having paired electrons within d orbital
some bonding geometries require hybridization that favors low-spin configuration
Iron exhibits different spin states
Under crustal conditions, high-spin configuration dominates in most minerals
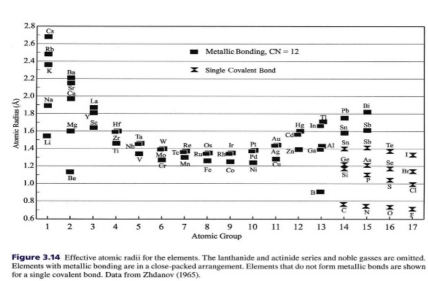
The End